Cell therapy and gene therapy are cutting-edge approaches in modern medicine, aiming to treat or potentially cure diseases at a fundamental level. Cell therapy involves transplanting healthy or modified cells into a patient to repair or replace damaged tissue, and it is commonly used in regenerative medicine and cancer treatments.
In contrast, gene therapy alters or replaces faulty genes within a patient’s cells to correct genetic disorders or stop disease progression. While both therapies have transformative potential, they differ in their mechanisms—cell therapy focuses on replacing cells, while gene therapy targets the underlying genetic causes of diseases. Below is a brief comparison between cell therapy and gene therapy. (See the table below)
Table of Contents
Cell Therapy vs Gene Therapy: Comparison
Cell Therapy | Gene Therapy |
1. Cell Source: Cell therapies can use various types of cells, including: – Stem cells (pluripotent, multipotent, or tissue-specific) that can differentiate into specialized cell types to regenerate tissues. – Immune cells (e.g., T cells or NK cells) that are genetically engineered to attack cancer cells. – Autologous cells from the patient’s body or allogeneic cells from a donor. | Gene therapy is a cutting-edge treatment modality that aims to correct or modify genetic disorders at the molecular level. It involves introducing, modifying, or replacing genes in a patient’s cells to treat or prevent disease. Gene therapy can address inherited genetic diseases, some types of cancers, and certain viral infections by targeting the underlying genetic causes. |
How Cell Therapy Works: | How Gene Therapy Works: |
1. Gene Delivery: In gene therapy, a therapeutic gene is delivered to the patient’s cells, often using a vector (typically a virus) that efficiently transports the genetic material into cells. The most commonly used viral vectors include: – Adeno-associated viruses (AAV). – Lentiviruses. – Retroviruses. | 2. Delivery to Target Area: The cells are delivered to specific sites in the body (e.g., injected into tissues or infused into the bloodstream). In cancer therapy, for example, the cells may be engineered to target specific tumor antigens. |
2. Gene Editing: Some gene therapies involve directly editing the patient’s DNA using techniques such as: – CRISPR-Cas9: A precise and programmable tool used to cut DNA at specific locations to delete, replace, or repair defective genes at specific locations. – Zinc Finger Nucleases (ZFNs) and TALENs: Other gene-editing tools that can target specific genetic sequences for correction. | 3. Action in the Body: – Regenerative Mechanisms: In therapies using stem cells, the injected cells migrate to damaged tissues and differentiate into the necessary cell types, such as neurons, muscle cells, or heart cells, to repair and regenerate tissue. – Immunomodulatory Mechanisms: In therapies like CAR-T cell therapy, the patient’s T cells are extracted, genetically modified to express a receptor that recognizes cancer cells, and re-infused into the body. These engineered T cells bind to and kill the cancer cells. – Anti-inflammatory Mechanisms: Certain cell therapies target immune regulation by delivering cells that reduce inflammation, modulate immune responses, or promote tissue healing in autoimmune diseases. |
3. Types of Gene Therapies: – Ex vivo gene therapy: Cells are taken from the patient, modified in the lab, and reintroduced into the patient’s body. – In vivo gene therapy: The genetic material is delivered directly into the patient’s body, where it reaches the target cells. | 1. Correcting Mutations: – In gene therapy for genetic disorders, the introduced therapeutic gene replaces a faulty or missing gene responsible for the disease. For example, in spinal muscular atrophy (SMA), a missing or mutated SMN1 gene causes muscle wasting, and gene therapy can deliver a functional SMN1 gene to restore normal function. – Gene replacement: When a faulty gene causes disease, gene therapy can deliver a standard copy of that gene to restore normal protein production. |
Mode of Action in the Human Body | Mode of Action in the Human Body |
1. Stem Cells: – Tissue Repair: Stem cells can repair damaged tissue by differentiating into the needed specialized cells (e.g., cardiomyocytes in heart failure or beta cells in diabetes). – Paracrine Signaling: Stem cells also release bioactive molecules that stimulate endogenous cells to repair or regenerate tissue. | 2. Immunotherapy (CAR-T, TCR-T): – Targeting Tumor Cells: CAR-T cells are modified to express chimeric antigen receptors (CARs) targeting cancer cells. Once these CAR-T cells recognize the cancer cell antigen, they trigger an immune response that destroys the cancer cells. – Cytotoxic Effects: After recognizing their target, these engineered immune cells release cytokines and cytotoxic granules, directly killing tumor cells. |
2. Silencing Disease-Causing Genes: – In cases where a specific gene is overactive or harmful, gene therapy can use techniques like RNA interference (RNAi) to “silence” or reduce the expression of that gene. This can be useful in conditions where excessive protein production contributes to disease, such as certain cancers. | 3. Enhancing Immune Responses (Gene-Modified Immunotherapy): Immunotherapytreatment, gene therapy can modify a patient’s immune ceImmunotherapy recognizes and attacks cancer cells. A prime example is CAR-T cell therapy, where T cells are genetically engineered to express receptors that target and destroy cancer cells more effectively. – Cytokine enhancement: Gene therapies can also introduce genes that encode immune-boosting proteins like cytokines, improving the immune system’s ability to fight off cancer cells or viral infections. |
4. Restoring Missing Proteins: – Some diseases are caused by the body’s inability to produce essential proteins. Gene therapy can deliver a functional gene that encodes for the missing protein. For example, in hemophilia, gene therapy can introduce a working version of the gene responsible for producing clotting factors, reducing patient bleeding episodes. | 3. Enhancing Immune Responses (Gene-Modified ImmunotheraIn immunotherapy treatmenttment, gene therapy can modify a patient’s imimmunotherapyerapy recognizes and attacks cancer cells. A prime example is CAR-T cell therapy, where T cells are genetically engineered to express receptors that target and destroy cancer cells more effectively. – Cytokine enhancement: Gene therapies can also introduce genes that encode immune-boosting proteins like cytokines, improving the immune system’s ability to fight cancer cells or viral infections. |
Delivery Methods Gene therapy depends on how effectively the therapeutic gene reaches the target cells. There are several methods for gene delivery: – Viral Vectors: These modified viruses can carry therapeutic genetic material into a patient’s cells. These vectors are engineered to be safe by removing their disease-causing components. – Non-Viral Methods: These include techniques like liposomes (fat-based particles that carry DNA into cells), nanoparticles, or direct injection of plasmid DNA into tissues. | |
3. Gene-Modified Cell Therapies: – Targeted Gene Expression: In some cases, cells are genetically engineered to correct genetic defects or overexpress therapeutic proteins to address diseases like hemophilia or muscular dystrophy. | |
Delivery Methods The success of cell therapy largely depends on how effectively the therapeutic cells reach the target tissues. There are several methods for cell delivery: – Intravenous (IV) Infusion: Cells are delivered into the bloodstream, and this method is commonly used for systemic treatments like CAR-T therapies for cancer. – Intramuscular Injection (IM): Cells are injected directly into the muscle, and this technique is often used in regenerative medicine for localized tissue repair. – Intrathecal Administration: Cells are injected into theerebrospinal fluid and used to treat neurological conditions by targeting the central nervous system. – Intra-articular Injection: Cells are delivered into joint spaces, and they are primarily used in therapies for joint diseases like osteoarthritis. – Intratumoral Injection: Cells are injected directly into tumors and used in cancer therapies to attack or modify tumor environments. – Subcutaneous Injection (SC): Cells are delivered just under the skin, and this technique is commonly used for therapies that stimulate systemic immune responses. | Challenges in Cell Therapy 1. Immune Rejection: Allogeneic (donor-derived) cells may trigger immune responses, leading to rejection. 2. Tumorigenicity: Stem cells, especially pluripotent ones, risk forming tumors if they grow uncontrollably. 3. Complex Manufacturing: Scaling up the production of cells for therapy in a consistent, high-quality manner is technically challenging. |
5. Correcting Cellular Function: – Gene therapy can correct cellular dysfunction by targeting mitochondrial disorders or correcting the expression of enzymes or proteins in the cerebrospinal fluid. For example, in Leber’s congenital amaurosis (inherited blindness), gene therapy introduces a correct version of the faulty gene into retinal cells, restoring vision. | Challenges in Gene Therapy 1. Immune Response: Since viral vectors are commonly used for delivery, the body’s immune system may recognize and attack these vectors, reducing the effectiveness of the therapy or causing side effects. 2. Targeting Specific Cells: The therapeutic gene must reach the correct type of cells. For example, gene therapy to treat blood disorders must target hematopoietic (blood-forming) stem cells. 3. Off-Target Effects: Especially with gene-editing technologies like CRISPR, unintended changes to other parts of the genome can lead to harmful mutations or unwanted consequences. 4. Long-Term Expression: Some gene therapies require long-lasting or even permanent therapeutic gene expression. However, maintaining stable gene expression over time remains a challenge in some cases. |
Due to their innovative and complex natures, the regulatory landscape for cell therapy and gene therapy is evolving rapidly. Each therapy type is subject to rigorous oversight by regulatory authorities, including the U.S. Food and Drug Administration (FDA), the European Medicines Agency (EMA), and other global regulatory bodies. These agencies ensure these advanced therapies’ safety, efficacy, and quality while balancing the need for expedited approvals for life-saving treatments.
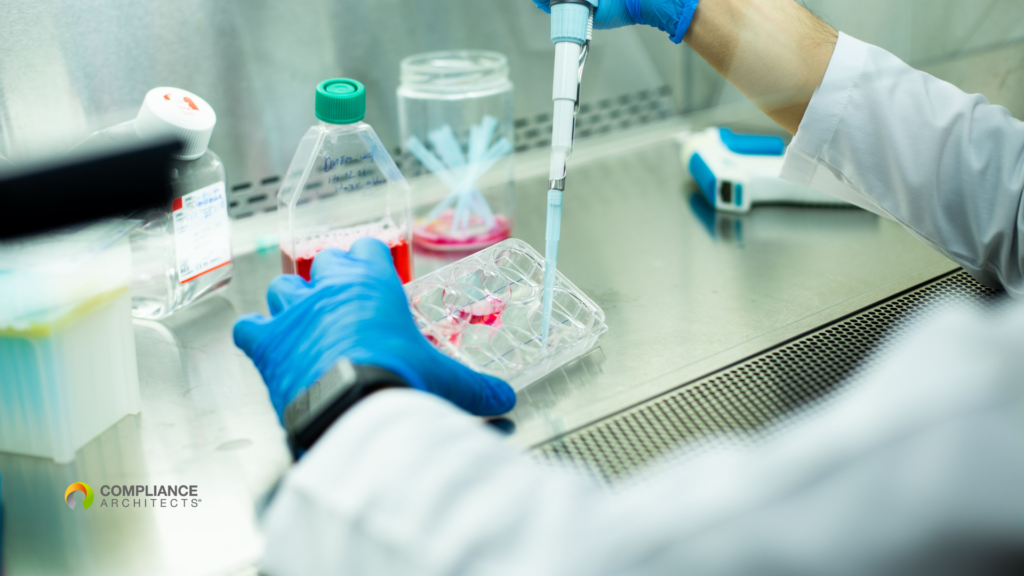
Common Regulatory Challenges
- Safety Concerns: Both cell and gene therapies face challenges related to safety, particularly immune reactions, off-target effects, and long-term risks. Regulatory bodies require extensive safety monitoring, both during clinical trials and post-marketing.
- Manufacturing and Quality Control: The complex manufacturing processes for cell and gene therapies require strict GMP standards and careful attention to consistency, reproducibility, and scalability.
- Expedited Pathways vs. Thorough Scrutiny: While expedited pathways are available to fast-track therapies with high unmet medical needs, balancing speed with the need for thorough safety and efficacy assessments is critical.
- Long-Term Monitoring: Due to the potential for delayed adverse events, both cell and gene therapies require long-term follow-up to monitor for any unforeseen risks, mainly as these therapies are often designed to have lasting or permanent effects.
Conclusion
The regulatory landscape for cell and gene therapies is continuously evolving to keep pace with their innovations and complexities. Global regulatory agencies such as the FDA, EMA, and PMDA provide multiple pathways to ensure these therapies are safe and effective while balancing the need for expedited access to life-saving treatments.
Contact Us
To learn more about cell therapy vs gene therapy, please get in touch with us by filling out the form below.